RESEARCH
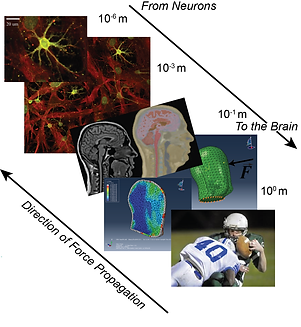
Development of a Predictive Multiscale Traumatic Brain Injury Model
(Multi-PI Initiative with Ron Szalkowski (Team Wendy), D. Hoffman-Kim, H. Kesari (Brown), L. Lamberson (Drexel), C. Hovey (Sandia))
Luke Summey, Jing Zhang
Successful protection and prevention of traumatic brain injuries requires a complete understanding of the small-scale, cellular response to the mechanical insult. Through extensive collaboration between our lab with Team Wendy, Drexel University, Sandia National Laboratories and the Office of Naval Research, this project establishes a comprehensive framework for identifying the onset of TBI in the brain and a new bottom-up approach for transformative material mitigation and brain treatment strategies.

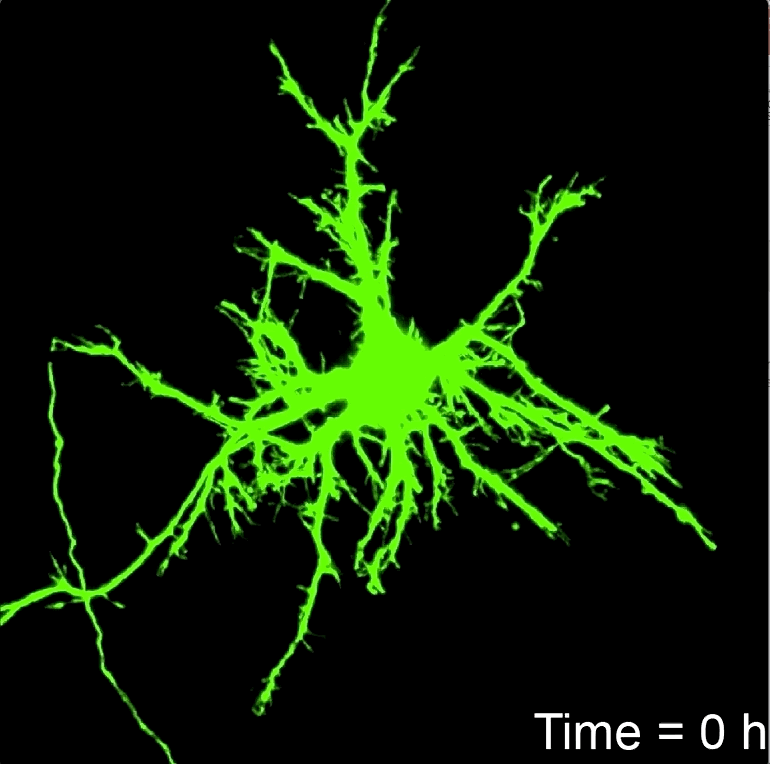
Experimental Characterization of Highly Compressible, Non-linear Viscoelastic Foams
(in collaboration with the Henann Group)
Light-weight, high-porosity (>80%) polyurethane foams are currently employed in many consumer applications from shoe insoles to soft body armor due to their exceptional energy dissipation potential. Yet their constitutive response remains poorly understood and poses a major obstacle in unlocking their true material potential.
Hypothesized to be a potential injury mechanism in blast and even blunt related traumatic brain injuries, inertial microcavitation is a powerful phenomenon capable of generating significant stresses and strains within brain tissue at hyper ballistic loading rates. This project aims to detail this new injury mechanism and its underlying neural pathology.
(Multi-PI Initiative with E. Johnsen, Z. Xu (Michigan), D. Henann (Brown), and T. Colonius (Caltech)
As opposed to traditional cavitation studies focused on understanding the physical behavior of expanding and collapsing bubbles in water or against metallic interfaces, this project focuses on understanding the physics of cavitation in complex soft materials. In particular, understanding how the rate-dependent, finite deformation behavior of length and time scale dependent compliant polymers affect the pressure, stress and strain fields in cavitating bubbles is investigated.
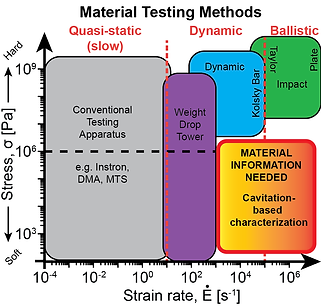

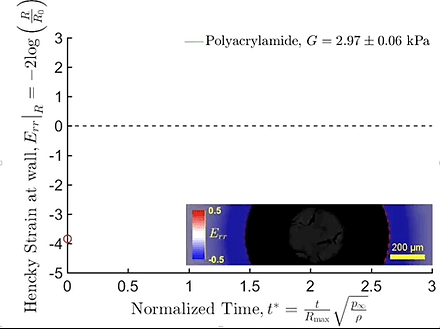
(in collaboration with Aviad Hai (Wisconsin) and Andrea Armani (USC))
The electrophysiology of neurons is important to understand as it contributes greatly to the function of the brain and the communication of the brain to different parts of the nervous system. This project aims to examine the change in neuronal activity during and after tension loading that simulates a traumatic brain injury. Using a fluorescent dye newly developed by the Armani Lab, this project will verify the accuracy of the dye as a way to obtain clear, meaningful electrophysiological data without the need for intracellular or extracellular electrical devices..
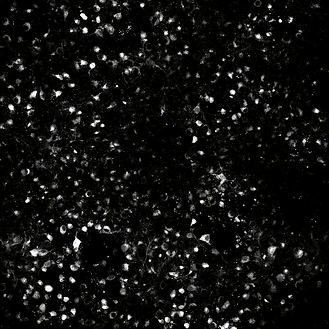
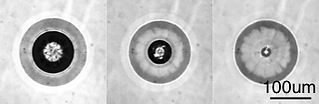
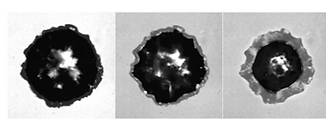
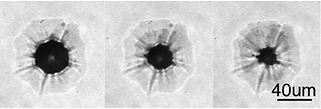
Characterizing material behavior is important for many applications because it provides a basic understanding of how that material will respond, given some deformation. This project aims to characterize the material behavior of porcine brain tissue that are undergoing local high-rate deformations and to also examine the spatial differences between anatomical regions of the porcine brain. This characterization will lead to a better understanding of the underlying behavior of the material, while providing data that could potentially be used to inform TBI models in a future project.
This project aims to characterize the high-rate, nonlinear behavior of novel viscoelastic materials through the use of our recently developed inertial microcavitation rheology (IMR).
Digital image and volume correlation methods have become the industrial and academic standard in resolving full-field displacement, strain and general motion fields. Yet, significant challenges remain for resolving large deformations in either highly rotational motion fields or environments providing poor speckle content or image quality. To this end we are developing novel, hybrid, local-global digital volume correlation schemes for addressing these more complex motion and sample scenarios.
Similarly, many inhomogeneous deformation fields such as generated by locomotion cells or cavitation bubbles require resolution of high spatial frequency content. Due to the intrinsic low-pass filter characteristics of correlation-based techniques, single particle tracking schemes have the ability to provide superior motion field reconstruction. Topology-based single particle tracking algorithms that feature multi-dimensional feature vectors can provide significantly improved resolution over traditional single particle, particle image velocimetry or digital image/volume correlation techniques, and is a focus of our lab.
Using these techniques, we continually update our 3D traction force microscopy algorithms accounting for large and viscoelastic deformations using both conventional epi-fluorescent as well as advanced (e.g., confocal, multi photon, super resolution) techniques.
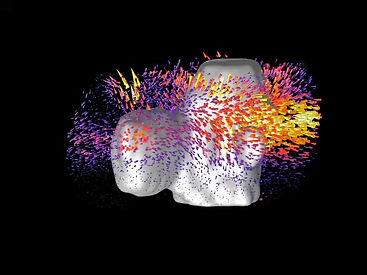
3D displacement field measured via our high-resolution topology-based single particle tracking (TPT) algorithm (Leggett et al., PNAS, 2020)
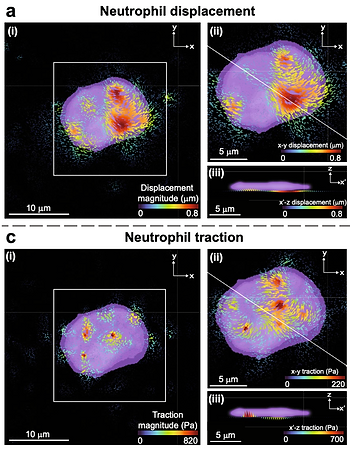
(Hazlett et al., Sci.Rep., 2020)
All of our codes providing both state-of-the-art image/volume correlation and single particle tracking motion reconstruction capability are freely available for download at our Github repository